Types of Fracturing Fluids
Most fracture treatments employ water-base fluids, both for safety reasons and for their relative ease of use. Oil-base and other fluids may be used when local availability and formation compatibility are primary considerations. In addition, compressed or liquefied gases such as nitrogen, carbon dioxide, liquefied petroleum gases (LPG), or liquefied natural gas (LNG)—alone or in mixtures with liquids and additives—are used for specific applications.
Water-Based Fluids
Water is used as a fracturing fluid in a wide range of formation types and over a wide range of temperatures and pressures. Compared with other fluids, it is generally inexpensive, readily available, and easy to modify with respect to fluid properties.
Viscosified Water-Based Fluids
It is often necessary to increase the viscosity of a water-based fracturing fluid to enhance its fracture propagation and proppant transport capabilities. Gelling agents commonly used as viscosifiers include:
- Natural guar gum (Guar)
- Hydroxypropyl guar (HPG)
- Hydroxyethyl cellulose (HEC)
- Carboxymethyl hydroxyethyl cellulose (CMHEC)
Natural guar gum is a chemical product processed from the commercially grown guar bean. During the refining process, some of the insoluble husk may become mixed in with the desired end product. This insoluble material, which can account for about 9 to 13 percent of the total solids content, can result in detrimental effects on proppant conductivity.
Hydroxypropyl guar (HPG) was developed as an alternative to natural guar gum to provide lower insoluble solids content. This manufactured material has a total insoluble solids content accounting for less than three percent of its total weight. The viscosity development and friction loss properties of natural guar and HPG are about the same. Most water-based fracturing fluid systems use one of these two viscosifiers.
Hydroxyethyl cellulose (HEC) and carboxymethyl hydroxyethyl cellulose (CMHEC) contain less than one percent insoluble materials. They are used infrequently because of certain temperature limitations, higher cost and, in some cases, the lack of supplemental fluid-loss control that an increased percentage of insoluble materials can provide. These fluids have been used extensively in high-permeability formations where fluid-induced formation damage is a primary concern. It is questionable as to whether or not these fluids are indeed less damaging, because the polymer penetration distance is larger than for wall-building fluids.
A linear gel viscosifier (one that does not incorporate crosslinking chemistry to link long polymer chains and create even greater viscosity) uses 10 to 100 pounds of gelling agent per 1000 gallons of water, with the usual concentration level ranging from 25 to 60 pounds per 1000 gallons. A typical HPG gel concentration of 40 lbm per 1000 gal of water contains 0.48 percent-by-weight of polymer. This concentration generally provides enough viscosity for the fluid to carry proppants through the surface equipment and tubular goods when pumped at a typical injection rate, although such a fluid might tend to allow proppant to drop out within the fracture at bottomhole conditions.
Crosslinked gel viscosifiers use similar polymer loadings, but develop considerably higher viscosities due to intermolecular bonding and the formation of a complex, three-dimensional network of long polymer chains (Figure 1). Through crosslinking, we can increase a fluid’s viscosity by several orders of magnitude while still maintaining its “pumpability.”
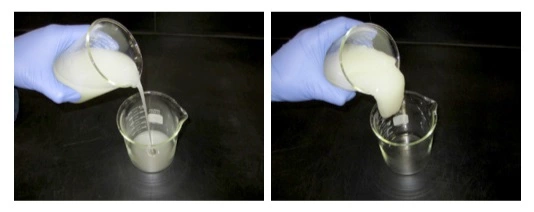
Crosslinking agents include borates and heavy metals such as zirconium and titanium. The crosslinker is normally added to the slurry after the base liquid has been blended with the proppant. Sometimes a delaying mechanism is incorporated using a crosslinking activator to prevent the crosslinking reaction from taking place until the fluid has reached the fracture. Most crosslinked fluids are extremely sensitive to temperature, pH and shear. The application of these sensitive crosslinking and associated delaying agents requires a precise control of the pH, accurate prediction of the temperature changes, and a comprehensive understanding of the shear history of the fluid as it moves through the system. Expected deterioration of viscosity due to shear in the tubulars can be countered with the use of delayed crosslinking agents. After a gel has delivered the proppant into the fracture it is necessary to “break” the gel to allow the polymer fluid to flow back into the wellbore.
Non-Viscosified Water-Based Fluids
“Slickwater” fracturing is the most basic and currently the most common form of well stimulation. In this application, the fracturing fluid is composed primarily (>98 percent) of water with chemicals added to reduce friction, inhibit corrosion and bacterial growth, and provide other benefits.
Low viscosity slickwater fluids generate fractures that are not as wide as high viscosity fluids. This means that there is more energy available to create greater fracture length and complexity, thereby connecting more of the reservoir to the wellbore.
Unfortunately, slickwater fracturing fluid is a poor proppant carrier. This means that high pump rates must be employed to achieve the high flow velocities needed to distribute the proppant before it can settle out. Slickwater fluids can be pumped down a wellbore as fast as 100 barrels per minute (bbl/min). The top speed of pumping without using slickwater is approximately 60 bbl/min.
Important benefits of slickwater fracturing are reduced gel damage, lower costs, higher stimulated reservoir volume, and better fracture containment within the formation. However, the process requires very large volumes of water, on the order of millions of gallons per treatment.
Gelled Oil-Based Fluids
Lease crude oil is still used for some fracturing treatments because of its relatively low cost and obvious formation compatibility. It is a very inefficient proppant transport medium and has poor fluid-loss control. But crude oil’s performance as a fracturing fluid, like that of water, can be improved with the use of additives. Fluid-loss additives can reduce the leakoff rate to reasonable values, and new generation viscosifiers allow proppant transport capabilities comparable with those of crosslinked water-based gels. The frictional pressure loss of gelled oil is much lower than that of un-gelled oil, in some cases approaching that of gelled water. Still, surface treating pressures for oils generally remain higher because the lower hydrostatic pressure of an oil column requires a greater surface pressure to achieve the necessary bottomhole fracturing pressure. Lease oil and gelled oils are used primarily in formations that are extremely sensitive to water. Occasionally, aluminum-phosphate-esters are used in oil-based fluids as viscosifiers and friction loss reducers.
Acid-Based Fluids
In limestone or dolomite formations, hydrochloric acid is sometimes used in conjunction with fracturing fluids to dissolve a portion of the rock, etching flow channels on the opposing fracture faces. This approach may be used in place of proppants; when the fracture faces close against one another the etched flow paths remain open. The resulting “fracture” conductivity can be quite high.
However, because of the rapid rate at which the acid is spent within the formation (especially at elevated subsurface temperatures), the use of acid-based fluids is limited to treatments in which only relatively limited horizontal fracture extension is required, or where the bottomhole temperature is relatively low. We can extend this technique’s applicability by using acid retarders, but the relatively high cost of acid versus proppants limits its potential economic benefit.
Alcohol-Based Fluids
In the 1990s some service companies used methanol as a base fluid in fracturing applications in Canada and Argentina, in cases where the fractured formations had low permeability with high clay content and/or low bottom-hole pressure leading to minimal fluid recovery (Gandossi, 2013).
However, methanol is used infrequently as a base fluid due to the risks and costs of handling methanol safely. The flash point (the lowest temperature at which it can vaporize to form an ignitable mixture in air) of methanol is 53°F (11.6°C) and its density is greater than that of air, allowing it to accumulate in low places if leaked and present a safety hazard to field personnel. In addition, methanol burns with an invisible flame. Compared to water-based fracture fluids, methanol-based fluids are 3 to 4 times as expensive.
Nonetheless, in formations with severe liquid (aqueous and hydrocarbon) trapping problems, non-aqueous methanol fluids may be a solution (Gupta, 2010). Advantages of alcohol-based fluids include low freezing point, low surface tension, high water solubility, high vapor pressure and formation compatibility. In underpressured wells, methanol can be energized with nitrogen or carbon dioxide. Methanol can be viscosified with crosslinked gels.
Methanol is widely used as an additive in hydraulic fracturing, for instance as a corrosion or scale inhibitor, friction reducer, formation water flowback enhancer and fracturing fluid flowback enhancer (Saba et al, 2012).
Gas, Liquefied Gas, and Gas-Liquid Foam Fluids
There are several types of hydraulic fracturing fluids that use little or no water and large amounts of nitrogen or carbon dioxide. The number of these “energized” fracturing jobs is relatively small compared to water-based treatments, representing less than 2-3% of the fracturing jobs in the U.S. and 20-30% of the fracturing jobs performed in Canada (Figure 2). Even though nitrogen and carbon dioxide based fracturing methods have been available since the 1970’s, they represent a niche portion of the market.
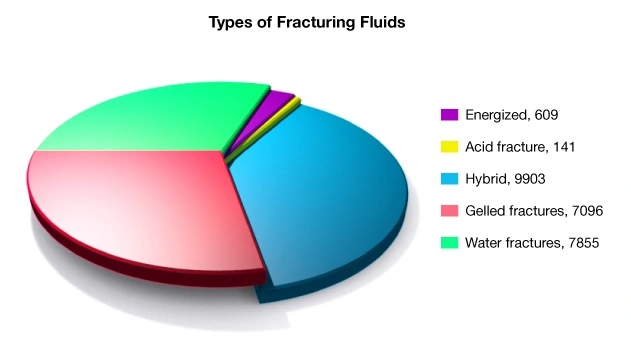
Nitrogen-Based Foam Fracturing
Nitrogen-based foam fracturing is a hydraulic fracturing method that contains only 8-25% water by volume, with the remaining volume occupied by nitrogen and foam-forming surfactants. It was introduced into North America in the early 1970’s by Nowsco Well Service of Calgary, Alberta. Foam fracturing is suitable for low pressure, tight gas formations that are sensitive to water.
Due to the exceptionally high horsepower required to pump this low-density fluid, foamed fluid systems are generally not considered for high pressure or deep reservoirs. The use of high-quality foam as a fracturing fluid is generally limited to wells shallower than 10,000 ft.
CO2-Based Foam Fracturing
CO2-based foam fracturing is similar to nitrogen-based foam fracturing, but employs compressed carbon dioxide rather than nitrogen. Either of these inert gases (N2 or CO2) can be used, depending on cost and availability. Recent increases in the price of carbon dioxide in the US and Canada has acted to dampen the use of this fluid by producing companies.
CO2-Sand Fracturing
CO2-sand fracturing is a fracturing process that uses only sand and carbon dioxide with no water. A closed system blender augers sand out of a pressure vessel pre-loaded with sand. The process was originally developed by Canadian FracMaster in the 1980’s. The sand is mixed and transported with liquid CO2 down the wellbore. The fact that the CO2 is pumped as a supercritical liquid rather than as a gas is important, as the liquid hydraulically creates the fracture and then vaporizes at reservoir conditions, leaving a liquid-free, residue-free sand-packed hydraulic fracture. The process is non-damaging to the reservoir compared to gel-based fracturing fluids, does not induce the relative permeability damage associated with capillary fluid retention in low pressure formations, and is non-damaging to water-sensitive clays that can plug pore throats and reduce permeability. The process has been used most successfully where formation damage near the wellbore needs to be overcome with small to moderate scale fracture lengths. However, application of CO2-sand fracturing appears to be limited due to concerns over CO2 availability and cost.
Straight Nitrogen or Straight CO2 Based Fracturing
A straight nitrogen or straight CO2-based fracturing is an alternative to water-based hydraulic fracturing where there are concerns of formation damage. In this case, nitrogen or carbon dioxide gas, or liquid carbon dioxide, is pumped without added liquid, surfactants or proppant. The application has been successful where horizontal stress differences may make proppant less important to fracture conductivity or where the process is being employed to remove liquid blockage from previous fracturing fluids and restore production. One example of this application is in the stimulation of horizontal wells in the Huron shale in West Virginia and Kentucky. The Huron shale is a low pressure shale gas reservoir where near-wellbore permeability can be reduced when water is used as a hydraulic fracturing fluid. The producer, EQT, found that straight nitrogen fracturing is superior to nitrogen foam fracturing in horizontal Huron wells (Wozniak et al., 2010).
Gelled LPG Fracturing
Gelled LPG fracturing is a process that uses gelled liquefied petroleum gas (LPG) and sand in a pressurized closed system blender. After the fracture is created the gelled liquid propane reverts to a gas and exits the well along with the natural gas or oil stream being produced, entirely eliminating the need for water to pumped, produced, or treated and disposed. This process does result in additional safety risks. Fracturing jobs are performed via remote control operation with no personnel on site.
LNG Fracturing
LNG fracturing uses liquefied natural gas as a large percentage of the fracturing fluid similar to how nitrogen or carbon dioxide is used in nitrogen-based foam fracturing or carbon dioxide-based foam fracturing. The smaller portion of the sand-laden fracturing fluid can consist of a variety of conventional oil and water based fracturing fluids. This process allows the flexibility of using all conventional oil and water based fracturing fluids on the sand blender side of the process which makes this process extremely versatile and open to operator-specific designs of fracturing fluids. This process can reduce the total water requirements to less than 10% by volume. During the flow back process the natural gas can be sold into the pipeline. Considerable oilfield service company investment in equipment is necessary. Other factors involve the demonstration of safe and remote execution of the process.
Emulsions
Oil and an aqueous mixture (water plus additives or an acid solution) are sometimes emulsified and the result used as a fracturing fluid. Such fluids can be economical alternatives, particularly when the cost of crude oil is low. The high viscosity of an emulsion creates wider fractures than does an aqueous linear gel, and assists in both proppant transport and reducing fluid leakoff. Emulsions are especially effective in controlling fluid loss because the fluid that leaks off from the fracture is a multiphase mixture. The relative permeability to a multiphase system is always lower than either single phase. Mixtures containing as little as 5% by volume of a second discrete fluid phase have been effective in limiting leakoff.
To minimize friction losses, emulsions having water as the outside phase are preferred, although emulsifiers that allow oil to form as the outside phase of the emulsion are sometimes used for special situations such as in preparing a liquid-encapsulated acid system (for retarding acid activity).