Source Rocks: The Origin of Petroleum
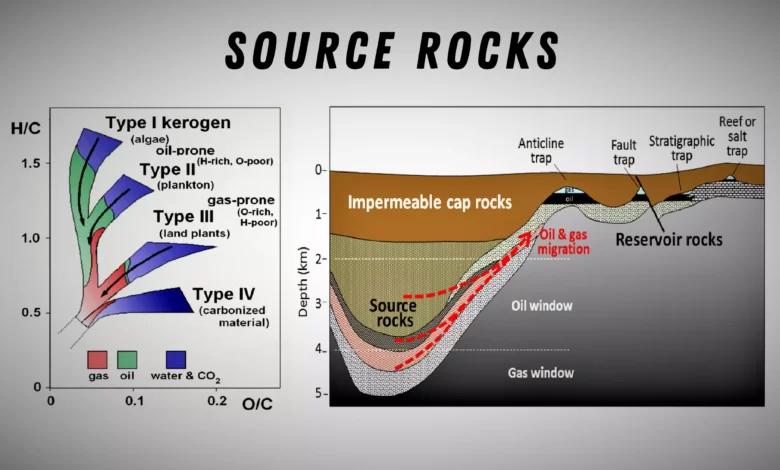
In the world of petroleum exploration, source rocks play a pivotal role in the formation and accumulation of hydrocarbon reserves. These organic-rich sedimentary rocks are the ancient repositories of organic matter that, under specific conditions, transform into valuable hydrocarbons. Understanding the significance of source rocks is crucial for successful exploration and exploitation of petroleum resources. In this article, we will delve into the depths of source rocks, exploring their formation, evaluation techniques, and their role in the creation of hydrocarbon reservoirs.
Introduction
Petroleum, often referred to as “black gold,” has been the lifeblood of modern industrialization. It fuels transportation, powers industries, and provides the raw materials for countless products. But where does petroleum come from? This is where source rocks come into the picture above.
Definition of Source Rocks
Source rocks are sedimentary rocks that contain abundant organic matter, capable of generating and expelling hydrocarbons over geologic time. They serve as the ultimate source of hydrocarbons, acting as natural factories that produce, accumulate, and release these valuable resources.
Formation and Preservation of Organic Matter
Prevailing evidence indicates that petroleum is derived from organic matter formed at the surface of the earth. Organic matter is a product of the highly selective carbon cycle (Figure 1), with degradation of plant and animal matter ultimately leading to concentrated accumulations of fossil fuels.
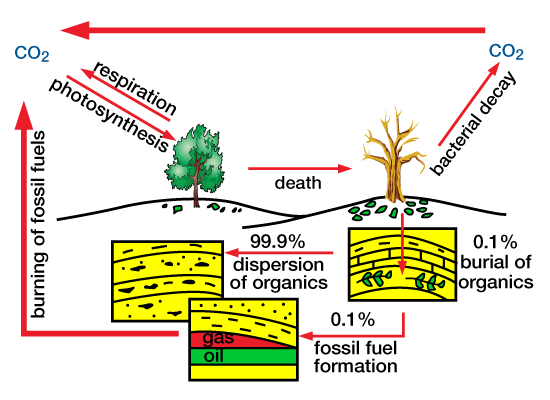
The carbon cycle begins with photosynthesis, a process that converts water and carbon dioxide in the atmosphere into glucose, water and oxygen. Glucose is a complex organic compound found in plants and in the animals that consume them. Most of this organic matter is recycled to the atmosphere as carbon dioxide through respiration or bacterial decay. Only one in 1000 parts of organic matter is buried rather than recycled. Over geologic time, this minuscule preservation accumulates to enormous amounts of fossil organics, estimated to be 20 × 1015 metric tons (Waples, 1981). Most of these organics are dispersed rather than concentrated in sediments, so approximately only one molecule of carbon dioxide in each million molecules produced by photosynthesis ever becomes part of an economic concentration of hydrocarbons.
Organic matter derived from plants and animals is concentrated in sediments by low- energy depositional environments. Organic matter settles out of suspension in quiet water, whereas it either stays in suspension or is winnowed by currents and waves. Therefore, organics tend to be more abundant in fine-grained sediments, such as muds and shales deposited in swamps, lakes and oceans.
In the oceans, concentrations of organics are closely related to production rates of microscopic floating plants such as diatoms and dinoflagellates, known as phytoplanktons, and blue-green algaes. Because of photosynthesis, light is important in the production of phytoplankton and algae. The photic zone defines the depth to which light penetrates below the ocean’s surface. The thickness of the photic zone is a function of latitude and water clarity. Productivity of organic matter is highest in clear, tropical seas where the photic zone often reaches water depths of 100 m.
The nutrient and oxygen supply to the photic zone is also important to organic productivity. The principal nutrients are phosphates and nitrates released by the bacterial decay of organic matter. As a by-product of photosynthesis, phytoplankton increase the oxygen content of surface waters. At the equator and at latitudes of about 60 degrees north and south, upward-circulating currents carry nutrients and dissolved oxygen from ocean depths to the surface, creating zones of high biological productivity. Other highly productive zones occur over the continental shelves in shallow, mixed waters, especially where rivers supply abundant nutrients to the ocean.
Quality source rocks require both high production and preservation of organic matter. Quick deposition and burial help to preserve organic material. However, bacteria in the sediments destroy organic matter. Aerobic bacteria use dissolved free oxygen in the overlying water to decompose most of the plant and animal remains at the sediment/water interface. At greater depths, anaerobic bacteria use fermentation to break down organics and produce biogenic methane. Bacterial action is generally limited to the upper 60 cm of sediment. Rapid burial, therefore, limits the time in which bacteria can act.
Preservation of organic matter is enhanced by stratified, oxygen-poor bodies of water, since a limited supply of oxygen will slow the loss of organic carbon. Density stratification of waters produces oxygen-deficient bottom layers. This phenomenon occurs in lakes, lagoons and shallow, restricted seas where fresh river water enters a body of dense salt water. Fresh water floats on saltwater, and the density contrast prevents oxygen produced by photosynthesis in the upper layer from circulating to the lower layer. Consequently, the oxygen supply is depleted at the bottom, where organic detritus settles. In the resulting anoxic environment, the decay rate of organics is slow because aerobic bacteria and sediment-eating organisms cannot survive. Anaerobic bacteria break-down organic molecules incompletely, thereby enhancing the transformation of organic matter to petroleum. Figure 2,
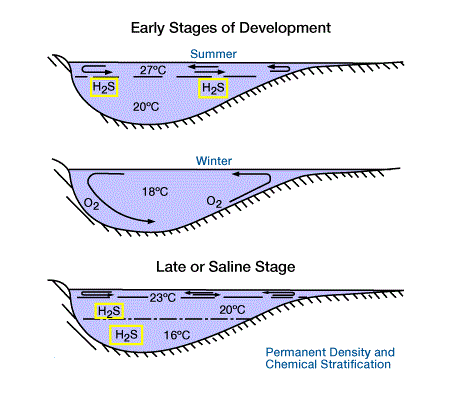
(Schematic model for stratified, oxygen-depleted lake, showing early, seasonal stratification, and permanent, late-stage stratification.) illustrates density stratification and oxygen depletion in inland seas, such as the Black Sea and the Dead Sea, and in lakes such as the Great Salt Lake of the western United States.
This density stratification also occurs on broad, flat shelves where circulation is minimal. In the oceans, a zone of oxygen depletion occurs at a depth of approximately 200 meters, with oxygen in greater abundance in both shallower and deeper waters (Figure 3). Eustatic rises in sea level can raise this anoxic zone enough to cover continental shelves, as was the case in Cenomonian time, when a maximal transgression created organic-rich shales worldwide.
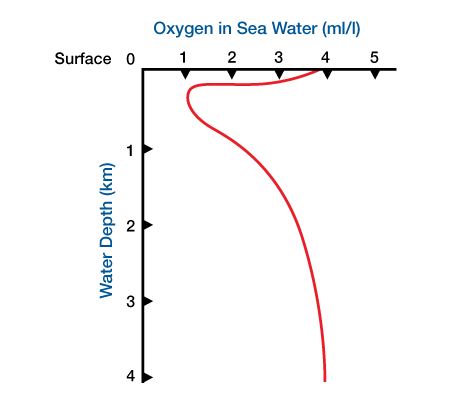