Layered Earth Structure
Let’s consider four variations of layering within the lithosphere. Each layer has different mechanical or thermal properties that influence the response of the lithosphere to stress.
The first factor in the formation of a basin is a layered earth structure. Figure 1 illustrates four different models of layers within the lithosphere.
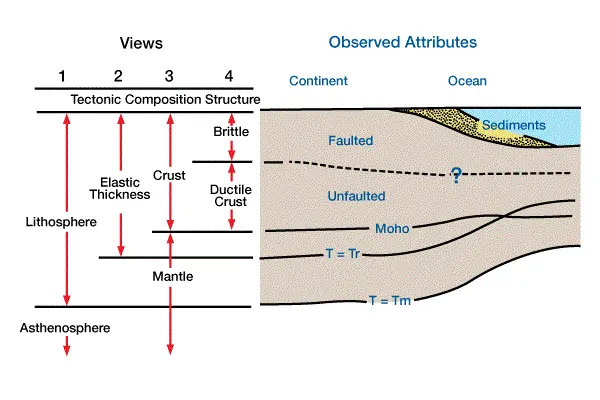
We recognize different types of layers using different criteria, depending on the nature of our interpretation problem. These layers have mechanical or thermal properties that influence the response of the lithosphere to stresses. The boundaries of the different types of layers commonly do not coincide. For example, layers defined by density differences do not necessarily correspond to thermal-rheological layers.
Layers in Views 1 and 2 are rheological layers that correspond to isotherms. In recognizing these layers, we estimate large-scale rheological properties within the earth and assume that these properties are controlled primarily by temperature. We use these layers to model basin formation and heat flow in response to tectonic, sedimentary and eustatic loads. The base of the lithosphere corresponds to a temperature Tm, the solidus temperature of the mantle , believed to be 1200 to 1350 degrees C. The lithosphere is composed of the crust and a portion of the mantle that is cooler than the solidus temperature Tm. We call mantle that is part of the lithosphere lithospheric mantle, subcrustal lithosphere or mantle lid . Above this isotherm, the lithosphere behaves like a solid on geologic time scales, but below this isotherm, the asthenosphere behaves like a low-viscosity fluid. Using this definition, the lithosphere is equivalent to the “plates” of plate tectonic theory.
View 3 shows layers based on composition and/or density. The crust-mantle transition marks either a mineralogical phase change or a compositional boundary. We recognize this transition, known as the Mohorovicic Discontinuity, or Moho , by a significant increase in seismic velocities. The compressional velocity in the mantle is typically about 1 km/sec greater than in the crust. We use the crust-mantle transition as an important boundary in gravity models for modeling density variations within the lithosphere.
In View 4, we divide the crust into layers that behave in brittle or ductile fashions. Above the brittle-ductile boundary, deformation produces brittle fracturing and faulting, while below this boundary, deformation results in ductile flow. We use this boundary to model structural styles as a response to regional tectonic stress. The effective elastic thickness , h, of the lithosphere is the thickness of earth that deforms elastically in response to loads. We define this thickness using an isotherm Tr, or the temperature of relaxation , of about 300 to 600 degrees C (Watts, 1978).
If the rheological layers of a basin correspond to the lithosphere and the elastic layer, then subsidence is controlled by the thicknesses of these layers. Because these rheological layers are defined by isotherms, the thermal structure of the lithosphere is a primary control on basin deformation. We use lithospheric age as a first approximation of lithospheric thickness when estimating response to loads, unless heat has been added by a thermal event since basin formation, or unless the rate of cooling is altered because of the geometry of the basin. For example, the flanks of a narrow rift basin can act as heat sinks to increase the rate of cooling. Consequently, the lithosphere would be cooler, thicker and more rigid than we would estimate solely from its age.