Reservoir Description and Modeling
Determination of hydrocarbon in place, reserves, and production potential requires an accurate physical description of the reservoir. The basic elements of such a description are depicted in Figure 1.
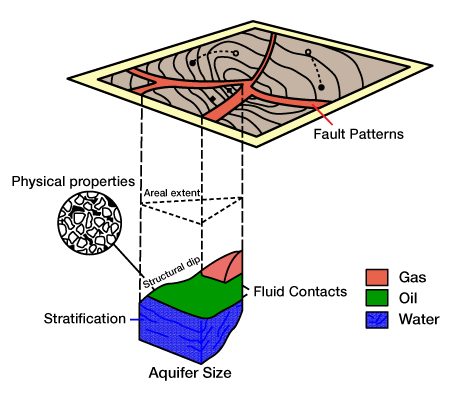
Areal Extent
The area of the reservoir is needed for calculating the hydrocarbon in place, for selecting the proper locations of wells, and as input data for reservoir simulation studies.
Physical Properties of the Productive Formation
Physical properties include formation thickness, porosity, water saturation, and permeability. These four parameters are needed in practically all aspects of reservoir engineering calculations. Preparation of contour maps for these properties constitutes the first and most important step in preparing a data base for reservoir engineering calculations.
Structural Dip
Reservoirs with a high angle of dip are good candidates for gravity drainage production. For secondary recovery projects in such reservoirs, one locates water-injection wells downdip and gas-injection wells updip for maxi mum recovery. Thus the angle of dip is an important factor in formulating a recovery plan.
Continuity of Strata and Stratification
Continuity or lack of continuity of the productive zone determines the pattern of depletion. Identification of separate zones or communicating zones, and the degree of communication, is necessary for establishing the optimum number of wells during primary production and EOR operations.
Fault Patterns
The location of faults and their effects as barriers to flow define the boundaries of the reservoir and help determine the locations of production and injection wells. Fault patterns strongly affect the design of the field development plan. The number and orientation of faults strongly influence the number of wells (and, in the case of offshore, the number of platforms) required for development.
Fluid Contacts
Determinations of oil-gas, oil-water, or gas-water contacts are needed for a complete description of the reservoir. Without such information, the hydrocarbon in place cannot be determined to a reasonable degree of accuracy and a proper recovery plan cannot be developed.
Aquifer Size
The size of the aquifer relative to the hydrocarbon reservoir is important in predicting recovery under primary depletion. Furthermore, this measurement has a strong bearing on the planning of a secondary or tertiary operation.
Reservoir Models
Reservoir engineering calculations require the formation of a mathematical model for the reservoir. This model should be based on the physical model that emerges from data obtained from the geological, geophysical, petrophysical, and log information. It is evident that in the majority of reservoirs the complexity is so great that it is not practical to expect a faithful mathematical description. Furthermore, it is impossible to obtain a physical description of the reservoir that is 100 percent accurate. One knows the physical properties of the reservoir to a high degree of accuracy only at well locations. In between the wells, or in the part of the reservoir for which no subsurface data are available, the physical description can only be deduced. The more drilling, the better the definition of the reservoir.
However, 3-D seismics and cross-well seismic tomography can provide information about the portions of the reservoir that lie between wells. 3-D seismics employs large amounts of closely spaced data and improved migration techniques to provide volumetric reservoir interpretations, while cross-well tomography apples high-frequency seismic waves, in which both source and receiver are located in existing wellbores. These tools give the geophysicist an active role to play in modeling the reservoir.
The mathematical representation of the reservoir can range from a very simple model, the tank-type (or zero-dimensional) model, to a highly complex set of equations that require numerical techniques and computers for their solution (the reservoir simulation approach). In the tank-type approach, the engineer assumes that the reservoir can be described with average values for properties such as thickness, porosity, and fluid saturations. While this approach may be satisfactory for simple problems, it may not be sufficient for other purposes. For instance, the tank-type model or a variation of it is normally used in volumetric estimation of the initial oil or gas in place. In some reservoirs it may also be satisfactory for material balance calculations. However, in other reservoirs such a model might be totally unsatisfactory and the engineer would have to resort to reservoir simulation. Generally speaking, as the heterogeneity of the reservoir in creases, so too does the required complexity of the mathematical representation.
Reservoir Simulation
As the complexity of a reservoir increases, the need for a more complex mathematical representation arises. The engineer must use a reservoir simulator to predict the performance of the reservoir under various development schemes.
Modern reservoir simulation is based on the tank type model, which forms the basis of reservoir engineering. However, rather than considering the reservoir as one tank unit, the simulation divides the reservoir into many tank units that interact with each other. The number of tank units, or cells, depends on many factors,including the heterogeneity of the reservoir, the number of wells, and the field development scheme. Heterogeneous reservoirs require a larger number of cells.
The basic reservoir engineering equations that have been used to describe the reservoir when represented by one tank unit are used in reservoir simulation. In the single-cell representation, no oil or gas crosses the boundary of the tank (i.e., reservoir). However, in a simulation with many cells, each cell interacts with its neighbors. Fluids may enter a cell from adjacent cells or may leave a cell and go to the cell’s neighbors.This fluid movement is governed by a well established flow equation, known as Darcy’s law. Keeping an inventory of the fluids in each cell is a rigorous bookkeeping operation, well suited to computers. The advent of the modern computer has increased the reservoir engineer’s simulation capabilities.
The rock and fluid data required for reservoir studies using the one-tank model representation are required for each unit cell in a simulation study. The effort required to prepare such data and input it to the simulator is a significant part of the cost, which can range from tens to hundreds of thousands of dollars, depending on the size, complexity, and purpose of the model.
Reservoir Boundaries and Heterogeneities
Boundaries
A reservoir may have closed or open boundaries, or both. If the reservoir is completely bounded by sealing faults or pinchouts, it is closed. Some reservoirs are completely surrounded by an aquifer, thus their boundaries are open to water movement into the hydrocarbon zone. Still other reservoirs may be bounded by faults or pinchouts along part of their boundary and by an aquifer along the remaining part. Most reservoir engineering calculations require an accurate knowledge of the boundary conditions of the reservoir. This knowledge may establish the possible existence and extent of an aquifer activity in the reservoir.
Heterogeneities
All reservoirs are heterogeneous, varying only in their degree of heterogeneity. This means that the physical properties of the rock change with a change in location. One of the very important heterogeneities that needs to be considered in reservoir engineering calculations is stratification. Many reservoirs contain layers (strata) of productive rock that can be communicating or non communicating. These layers can vary considerably in permeability and in thickness. A good description of the layers and their respective properties is critical in planning many EOR operations.
Fault System
Another common heterogeneity in reservoirs is the fault system. Faults can be completely or partially sealing. Well locations for both production and injection are affected by the fault pattern and its effect on fluid communication. Faults are normally defined from geological, geophysical, and production data.
Permeability
Permeability is another directional property. When permeability measurements vary depending on the direction in which they’re measured, we say that the reservoir is anisotropic with respect to permeability. Permeability anisotropy is important in determining well spacing and configuration, as well as in considering the option of horizontal wells.